...::::: NOVELTIES
:::::.....
|

PÌ-303
PTA system for automated surfacing with CNC control
PM-WMO-120
Welding mechanical oscillator

PM-RM-100
Rotator-Manipulator

PP-7
Compact plasma torch for outer surfacing of small-sized parts

PM-PF-10
Powder feeder
|
...::::: EVENTS
:::::.....
|

|
...::::: ADVERTISING
:::::.....
|
|
 |
1 · · · 2
· · · 3
· · · 4 · · · 5
· · ·
|
|

|
Laser+plasma:
search for new possibilities in surfacing |
Article: Authors - Som À.I., Krivtsun
I.V.
The Paton Welding Journal - 2000. - ¹12. - 36-41.
More and more publications appear in a scientific
and technical literature about application of combined
processes of welding based on a combined use of a
laser radiation and electrical arc, including plasma
arc [1-7]. Such combination leads to the improvement
of a space stabilization of an arc spot at the surface
of metal being welded and to the increase in stability
of its burning at low currents and high rates of displacement
relative to the work piece. Simultaneously, the coefficient
of absorption of a laser radiation by the metal surface
is increased that is especially important when the
low-power lasers are used. All this provides, from
the one hand, the significant increase in productivity
and stability of arc (plasma) welding, and from the
other hand, the increase in effectiveness and decrease
in the cost of the laser welding.
Our work was aimed at the conductance of theoretical
and experimental investigations of effect of a laser
beam on the process of a plasma-powder cladding (PPC)
and revealing of new technological capabilities of
a combined method, i.e. laser-plasma powder cladding
(LPPC). The most rational scheme of realization of
this combined process is, in our opinion, a coaxial
combination of a laser beam and plasma arc during
a distributed feeding of a filler powder into a discharge
plasma. As in this case the laser beam should pass
a definite distance in the arc plasma, then, during
development of the above-described method it is necessary
to take into account not only the plasma and laser
effect on the filler material and the surface treated,
but also the direct interaction of the focused laser
beam with a compressed arc plasma.
It is known that such interaction can lead to the
occurrence of a special type of a gas discharge, i.e.
a combined laser-arc discharge [5]. The necessary
condition of its occurrence is the commensurability
of energy, put into arc plasma by a laser radiation,
with energy, generated in the plasma at the expense
of the electric current passing. When this condition
is fulfilled, a significant change in energy balance
of the arc discharge takes place, thus resulting in
the fact that both integral and local characteristics
of the plasma of the combined discharge are differed
greatly from the corresponding characteristics of
the initial arc plasma. As to the characteristics
of the laser beam, then they undergo the significant
changes due to absorption of beam and its additional
focusing in the plasma of the laser-beam discharge.
Unfortunately, it is almost impossible to use the
traditional arc plasma torches for the creation of
a combined discharge and its use for LPPC according
to the above-described scheme. The coaxial combination
of the laser beam with a plasma arc requires the creation
of special devices, i.e. laser-arc plasma torches
[8-10]. These devices are distinguished mainly by
a design of the cathode unit (refractory tubular cathode
or a system of pin cathodes located around the circumference).
This peculiar feature makes it possible to introduce
a focused laser beam to arc plasma along the axis
of the plasma-shaping channel.
For a practical realization of the LPPC process and
revealing the technological capabilities of a combined
use of the plasma arc and laser radiation, a prototype
of a specialized laser-arc plasma torch LPP-22
was designed and manufactured (Figure 1). During its
design a large experience of Plasma-Master Co., Ltd.
on the creation of plasma torches for PPC [11], and
also results of theoretical studies of a combined
laser-arc discharge and devices for its realization,
made at the E.O. Paton Electric Welding Institute,
were used [5, 9].
|
a
Fig. 1. Appearance (a) and scheme of a nozzle
part (b) of the laser-arc plasma torch LPP-22
|
 |
|
In this plasma torch (Figure 1, b) the DC arc is
burning in an axial flow of the plasma gas (argon)
between the refractory (tungsten) tubular cathode
/ and the workpiece being subjected to cladding (anode)
2. At initial region a discharge is stabilized by
a wall of the plasma-shaping nozzle 3, which is coaxial
with a cathode. The nozzle 4 serves for a distributed
feeding of the filler powder to the discharge. Both
nozzles are made from copper and cooled by water 5.
The beam 6 of a continuous CO2-laser radiation, focused
by an optical system and spreading along the plasma
torch axis, is introduced to the discharge through
the cathode orifice. The plasma gas 7, 8 is supplied
to a plasma-shaping channel both through the cathode
orifice and to a gap between the cathode and a wall
of the nozzle 3. The gas consumption here may vary
independently of one another. The filler powder is
fed by a flow of the transporting gas 9 (argon) to
the gap between nozzles 3 and 4. The cladding zone
is protected by an argon flow. To protect the laser
focusing system in plasma torch LPP-22 an additional
gas gate is provided, and also appropriate correctors
of the cathode positioning relative to the beam axis
are provided to provide the coaxiality of a laser
beam and a plasma arc.
The plasma torch was designed for operation at the
arc current of 100 A < I < 300
A using a laser beam having a mode TEM 2.0, power
Qo<= 5 kW and angle of beam focusing
0.053. To define the optimum conditions of the LPP-22
plasma torch operation, characteristics of the generating
plasma and an interacting laser beam, a detailed computer
modelling of a combined discharge generated by the
mentioned plasma torch without taking into account
the feeding of the filler powder was made. Here, the
following sizes of the nozzle 3 were used (Figure
1, b): 4.5 mm length of a cylindrical channel (Ln1
= 5.5 mm), radius Rn1 = 5.0 mm. Respectively,
for nozzle 4, the length of the outlet channel is
2.5 mm (Ln2 = 10.0.mm), radius Rn2
= 5.0 mm. Consumption of the plasma gas, supplied
through the cathode orifice is G1 =
0.5 L/min, while between the cathode and the channel
wall it is G2 = 2.5 L/min. Consumption
of transporting gas was 5.0 L/min, and its initial
temperature was taken equal to the temperature of
water-cooled walls of the channels (300 K). Distance
f from the cathode edge till the focus
of the initial beam was varied within the range of
14 - 22 mm, and the length d of the
open region of the discharge was 4-12 mm.
|

Fig.2. Space distribution of temperature of
discharge plasma in laser-beam plasma torch
(I=200A, d=12mm, f=14mm) at laser beam power
1 (1), 2 (2), 3 (3). 0 kW (dashed curve)
|

Fig.3. Space distribution of gas-dynamic pressure
of plasma flow generated by laser-arc plasma
torch (conditions and designations are the same
as in fig.2.)
|
|
Calculations of characteristics of plasma and a laser
beam, interacting with it, were carried out on the
basis of a model of the combined discharge in a laser-arc
plasma torch, described in detail in [9]. Results
of numerical modelling of the discharge for different
conditions of the plasma torch operation are presented
partially in Figures 2-4. Thus, Figure 2 presents
space distributions of plasma temperature T, while
Figure 3 shows a distribution of gas-dynamic pressure
of its flow pu*u/2 (p
- density, kg/m3; u - rate, m/s). According
to curves given in Figure 2, the absorption of the
laser radiation by an arc plasma leads to a significant
increase in temperature of its central regions. Moreover,
the maximum achieved values T are increased
with a growth in beam power Qo. The
mentioned growth in the plasma temperature promotes
the increase in its electroconductivity and, consequently,
to the increase in current density in a near-axial
zone of the discharge. Thus, the discharge, generated
by a laser-arc plasma torch, is characterized by an
increased concentration of heat and electrical energy
in that region of the plasma, which is subjected to
the action of the laser beam and rigidly connected
with its axis and also by a high space stability of
this zone.
The described change in heat condition of the plasma
arc burning under the action of the laser radiation
causes a significant redistribution of gas-dynamic
characteristics of the plasma flow with increase in
Qo. One of the main causes is the decrease
in a viscosity of the argon plasma with the temperature
increase. Another cause is the above-mentioned redistribution
of current density in the discharge, thus increasing
the role of electromagnetic forces in accelerating
of the plasma flow [5].
As a result, the axial component of the plasma rate
at the discharge axis is increased noticeably. In
spite of increase in rate, the decrease in plasma
density at the temperature increase leads to the fact
that the gas-dynamic pressure of the plasma flow pu*u/2
in a near-axial zone of the combined discharge is
somewhat decreased (Figure 3). It should be noted
that this decrease, which causes the dynamic action
on the surface of the molten metal, is important for
the cladding process using a laser-arc plasma torch
[12].
|

Fig.4. Distribution of intensity of laser radiation
along the axis of a combined discharge (Qo=3kW, d=12mm,
f=14mm) at arc current 100 (1), 200 (2), 300 A (3),
dashed curve - without plasma arc
|
The interaction of a laser
radiation with an arc plasma, proceeding in the above-described
plasma torch leads to the redistribution of not only
its characteristics but also the laser beam itself (due
to absorption and refraction in the discharge plasma).
Thus, for example, the absorption of the laser radiation
leads to the fact that at the distance of 20 mm from
the cathode edge the beam power for the mentioned conditions
is only about 30 % of its initial power Qo,
whereas the intensity of radiation, Po,
at its axis increases by more than twice (Figure 4). |
Thus, the interaction of the laser beam with plasma
of a combined discharge causes its additional focusing,
which is increased with a growth of I
and Qo. Consequently, by varying these
two parameters, it is possible to control effectively
the beam focusing in plasma of the combined discharge
created with the help of a laser-arc plasma torch
that is important when similar devices for welding
and cutting are used.
Experimental investigations of the plasma torch LPP-22
were performed in Fraunhofer Institute of Technology
(Germany) using CO2-laser RS-5000 and plasma arc power
source Messer Griesheim Uniting GW 30. The plasma
torch was fastened to a focusing system by a special
adapter which makes it possible to coincide the axis
of the laser beam with the plasma torch axis. The
movement of the workpiece to be treated with respect
to the plasma torch was realized by a programming
welding manipulator.
Experiments were performed in two stages. First, the
peculiarities of burning the combined discharge without
feeding the filler powder were studied, then the laser-plasma
cladding of steel samples at different conditions
was performed. During all the experiments the arc
current was varied in the range of 100 - 280 A, the
laser beam power - 0 - 4 kW. The distance f
was changed within 14 - 22 mm, and d
(from the plasma torch edge to the anode-workpiece
surface) was set equal to 4, 8 and 12 mm. Consumptions
of transporting and plasma gas were constant and corresponded
to the above-mentioned values.
|

Fig.5. Dependence of discharge voltage in laser-arc
plasma torch (d=8mm, f=16mm) on initial beam power
Qo(a) and plasma arc current I (b): "black square"
- Ia=150, "dagger" - 200, "triangle"
- 250A; "rhomb" - Qo=0, "white square"
- 1, "circle" - 3kW; dashed line - calculated
data at I=200A
|
The experiments showed that the plasma torch LPP-22
operated with a high space-time stability of parameters
of the generating plasma within the entire examining
range of arc current and laser beam power. Volt-ampere
characteristics of discharge using a copper water-cooled
anode at different values Qo and I
were measured (Figure 5). The experimental data confirm
the theoretical results [5]: under the action of the
laser beam the arc voltage is decreased, and, as follows
from Figure 5, a (solid curves), its main drop occurs
at the laser power Qo < 2.5 kW. In
the same Figure the calculated dependence (dashed
curve) of a full voltage of discharge on the beam
power demonstrates quite satisfactory its coincidence
with experimental values. As to the plasma arc itself,
then under the action of the laser radiation it is
somewhat compressed that can be observed visually
or from the rising volt-ampere characteristics of
the discharge (Figure 5, b).
Investigation of technological capabilities of the
plasma torch LPP-22 was performed on flat samples
made from 20 mm thick low-carbon steel using LPPC.
Powdered alloys of Hastelloy C and Stellite 6 grades
of 20 - 63 mkm fractions were used as filler materials.
These investigations showed that the coaxial combination
of the plasma arc with a laser beam in the laser-arc
plasma torch gives an opportunity to increase the
speed of cladding of single beads by 2 - 3 times as
compared with conventional PPC due to improving the
space stability of the arc burning. This is similar
to the increase in productivity of cladding works
for such parts as screw conveyors of extruding machines,
flat and disc knives, milling cutters, etc. Simultaneously,
the melting y of the parent metal during cladding
of separate beads was at the level of 5 - 10 %. This
small value became possible due to the above-mentioned
dynamic action of the plasma flow to the melt surface.
In addition, the above-mentioned decrease in arc
voltage in the combined process reduces the risk of
a double arcing that is especially important in operation
at high currents (more than 300 A). This is a good
premise for increasing the cladding efficiency by
increasing the arc current. Unfortunately, it was
not possible to realize this opportunity in our series
of experiments, as the available power source could
not operate at currents of more than 300 A.
|

Fig.6. Appearance (a) of wide-layer laser-plasma
deposits 1, 2 and scheme of their cladding (b - 1,
c - 2) with appropriate conditions and macrosections
of transverse section of clad layers
|
Figure 6 presents two examples of a wide-layer LPPC,
made at Qo = 2 kW, 18 L/min, full gas
(argon) consumption and 2.7 kg/h powder (Hastelloy
C) consumption. In one case the cladding was performed
with transverse oscillations of the workpiece relative
to the plasma torch (Figure 6, b), and in the second
case - with longitudinal overlap beads (Figure 6,
c). In both cases a good formation of the deposited
layer was provided at minimum melting of the parent
metal (y < 5 %). This method
of surfacing opens up the feasibility of cladding
of large surfaces of the products using wear- and
corrosion-resistant alloys providing a high efficiency
and quality, for example, in production of bimetals.
For comparison, such efficiency of cladding (2.7 kg/h)
can be attained in case of using only a laser radiation
at the beam power of not less than 10 kW.
|

Fig.7. Macrosections of single beads at different
speeds of cladding: a - 12 (y=40%); b - 18 (y=15%);
c - 30 m/h (y=10%) at I=200 A, Qo=3kW, d=8mm,
f=16mm
|

Fig.8. Macrosections of single beads after
cladding at different powers of laser beam:
a - 0 (y=5%); b - 1 (y=10%); c - 4kW (y=10%)
at I=200 A, d=8mm, f=16mm, v=18 m/h
|
|
In the work, the effect of LPPC speed (Figure 7)
and laser beam power (Figure 8) on the formation of
single beads and also on the melting y
of the parent metal was also investigated. In experiments,
with increase of the cladding speed the section of
the beads tried to be kept constant by the appropriate
increase in the powder consumption.
As follows from the appearance of beads and macrosections
of their transverse section, a good formation of beads
is provided in the wide range of cladding speeds (from
10 to 50 m/h). In addition, the nature and level of
melting of the parent metal depends both on a total
power of the laser beam and plasma arc and also on
their ratio. With increase of the laser beam power
a peak of melting of the parent metal is shifted from
the edges of the bead to its axis (Figure 8). The
structure of metal deposited with LPPC method (Figure
9) is similar to that produced by using the conventional
PPC.
|
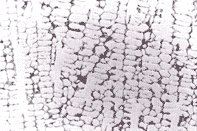
Fig.9. Microstructure of clad metal (Stellite 6)
(x500) (reduced by 2/3)
|
Thus, the combined application
of the laser beam and plasma arc opens up new possibilities
in cladding, consisting of increase in speed, stability
and reproducibility of the process results, reducing
in its cost, as well as in improving the use of the
laser energy. However, the authors understand that the
results given in this article are preliminary. |
For a practical realization of the combined process
the further investigations are required both from
the point of view of optimizing the process parameters
and also the selection of rational fields of its application.
CONCLUSIONS
1. Throughout the entire range of conditions the created
laser-arc plasma torch LPP-22 has a stable operation
with high space-time stability of parameters of the
generating plasma and can serve a prototype for making
the new designs of such devices for different technological
processes.
2. Interaction of the laser beam with a plasma arc
leads to the decrease in arc voltage that reduces
the risk of a double arcing.
3. It is possible to increase the speed of cladding
of single beads in the combined process by 2 - 3 times
as compared with a conventional PPC at the expense
of increasing the space stability of the arc.
4. The parent metal melting at the LPPC optimum conditions
can amount to 5 - 10 %.
The authors thank Dr.-Ing. T. Cheliker for participation
and assistance in conductance of the experimental
investigations.
|
REFERENCES
1. Steen, W.M. (1980) Arc augmented laser processing
of materials. /. ofAppl. Phys., 11, 5636 - 5641.
2. Diebold, T.P., Albright, C.E. (1984) "Laser-GTA"
welding of aluminium alloy 5052. Welding J., 6, 18
-24.
3. Matsuda, J., Utsumi, A., Katsumura, M. et al.
(1988) TIG or MIG arc augmented laser welding of thick
mild steel plate. Joining and Materials, 1, 31 - 34.
4. Walduck, R.P., Biffin, J. (1994) Plasma arc augmented
laser welding. Welding and Metal Fabrication, 4, 172
-176.
5. Gvozdetsky, V.S., Krivtsun, I.V., Chizhenko, M.I.
et al. (1995) Laser-arc discharge: theory and applications.
In: Welding and Surfacing Rev. Harwood.
6. Tusek, J. (1996) Sinergic operation of welding
arc and laser beam for practical application or for
scientific research only? Varilna Tehnika, 2, 39 -
46.
7. Dilthey, U., Lueder, F., Wieschemann, A. (1998)
Process-technical investigations on hybrid technology
of laser beam-arc welding. In: Proc. of 6th Int. Conf.
on Welding and Melting by Electron and Laser Beams.
Toulon, France.
8. Paton, B.E. (1995) Improvement of methods of welding
- one of the ways of improving quality and cost-effectiveness
of welded structures. Avtomaticheskaya Svarka, 11,3-11.
9. Krivtsun, I.V., Chizhenko, M.I. (1997) Fundamentals
of calculation of laser-arc plasma torches. Ibid.,
i, 16 - 23.
10. Dykhno, I.S., Krivtsun, I.V., Ignatchenko, G.N.
Combined laser and plasma arc welding torch. Pat.
5700989 USA, Int. Cl. B 23 K 26/00, 10/00. Publ. 23.12.97.
11. Som, A.I. (1999) New plasma torches for plasma-powder
surfacing. Avtomaticheskaya Svarka, 7, 44 - 48.
12. Krivtsun, I.V., Som, A.I. (1998) Modelling of
the laser-arc plasma torch. In: Proc. of 5th Int.
Conf. on Thermal Plasma Processes, St.-Petersburg,
Russia. New-York: Be-gelhouse.
|

1 · · · 2
· · · 3 · ·
· 4 · · · 5
· · ·
|
|